Publications
Efficient Computation of Shortest Path-Concavity for 3D Meshes
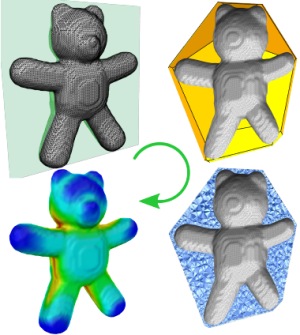
In the context of shape segmentation and retrieval object-wide distributions of measures are needed to accurately evaluate and compare local regions of shapes. Lien et al. proposed two point-wise concavity measures in the context of Approximate Convex Decompositions of polygons measuring the distance from a point to the polygon’s convex hull: an accurate Shortest Path-Concavity (SPC) measure and a Straight Line-Concavity (SLC) approximation of the same. While both are practicable on 2D shapes, the exponential costs of SPC in 3D makes it inhibitively expensive for a generalization to meshes. In this paper we propose an efficient and straight forward approximation of the Shortest Path-Concavity measure to 3D meshes. Our approximation is based on discretizing the space between mesh and convex hull, thereby reducing the continuous Shortest Path search to an efficiently solvable graph problem. Our approach works out-of-the-box on complex mesh topologies and requires no complicated handling of genus. Besides presenting a rigorous evaluation of our method on a variety of input meshes, we also define an SPC-based Shape Descriptor and show its superior retrieval and runtime performance compared with the recently presented results on the Convexity Distribution by Lian et al.
Practical Anisotropic Geodesy
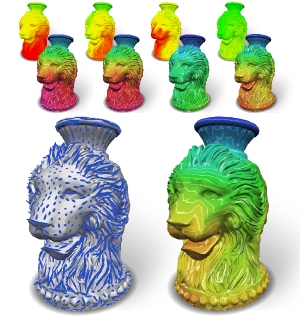
The computation of intrinsic, geodesic distances and geodesic paths on surfaces is a fundamental low-level building block in countless Computer Graphics and Geometry Processing applications. This demand led to the development of numerous algorithms – some for the exact, others for the approximative computation, some focussing on speed, others providing strict guarantees. Most of these methods are designed for computing distances according to the standard Riemannian metric induced by the surface’s embedding in Euclidean space. Generalization to other, especially anisotropic, metrics – which more recently gained interest in several application areas – is not rarely hampered by fundamental problems. We explore and discuss possibilities for the generalization and extension of well-known methods to the anisotropic case, evaluate their relative performance in terms of accuracy and speed, and propose a novel algorithm, the Short-Term Vector Dijkstra. This algorithm is strikingly simple to implement and proves to provide practical accuracy at a higher speed than generalized previous methods.
Walking On Broken Mesh: Defect-Tolerant Geodesic Distances and Parameterizations
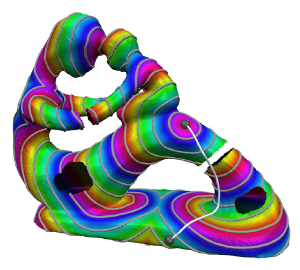
Efficient methods to compute intrinsic distances and geodesic paths have been presented for various types of surface representations, most importantly polygon meshes. These meshes are usually assumed to be well-structured and manifold. In practice, however, they often contain defects like holes, gaps, degeneracies, non-manifold configurations – or they might even be just a soup of polygons. The task of repairing these defects is computationally complex and in many cases exhibits various ambiguities demanding tedious manual efforts. We present a computational framework that enables the computation of meaningful approximate intrinsic distances and geodesic paths on raw meshes in a way which is tolerant to such defects. Holes and gaps are bridged up to a user-specified tolerance threshold such that distances can be computed plausibly even across multiple connected components of inconsistent meshes. Further, we show ways to locally parameterize a surface based on geodesic distance fields, easily facilitating the application of textures and decals on raw meshes. We do all this without explicitly repairing the input, thereby avoiding the costly additional efforts. In order to enable broad applicability we provide details on two implementation variants, one optimized for performance, the other optimized for memory efficiency. Using the presented framework many applications can readily be extended to deal with imperfect meshes. Since we abstract from the input applicability is not even limited to meshes, other representations can be handled as well.
Accurate Computation of Geodesic Distance Fields for Polygonal Curves on Triangle Meshes
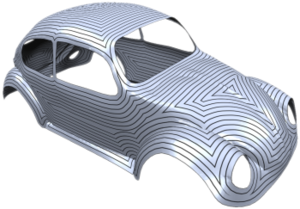
We present an algorithm for the efficient and accurate computation of geodesic distance fields on triangle meshes. We generalize the algorithm originally proposed by Surazhsky et al. . While the original algorithm is able to compute geodesic distances to isolated points on the mesh only, our generalization can handle arbitrary, possibly open, polygons on the mesh to define the zero set of the distance field. Our extensions integrate naturally into the base algorithm and consequently maintain all its nice properties. For most geometry processing algorithms, the exact geodesic distance information is sampled at the mesh vertices and the resulting piecewise linear interpolant is used as an approximation to the true distance field. The quality of this approximation strongly depends on the structure of the mesh and the location of the medial axis of the distance fild. Hence our second contribution is a simple adaptive refinement scheme, which inserts new vertices at critical locations on the mesh such that the final piecewise linear interpolant is guaranteed to be a faithful approximation to the true geodesic distance field.